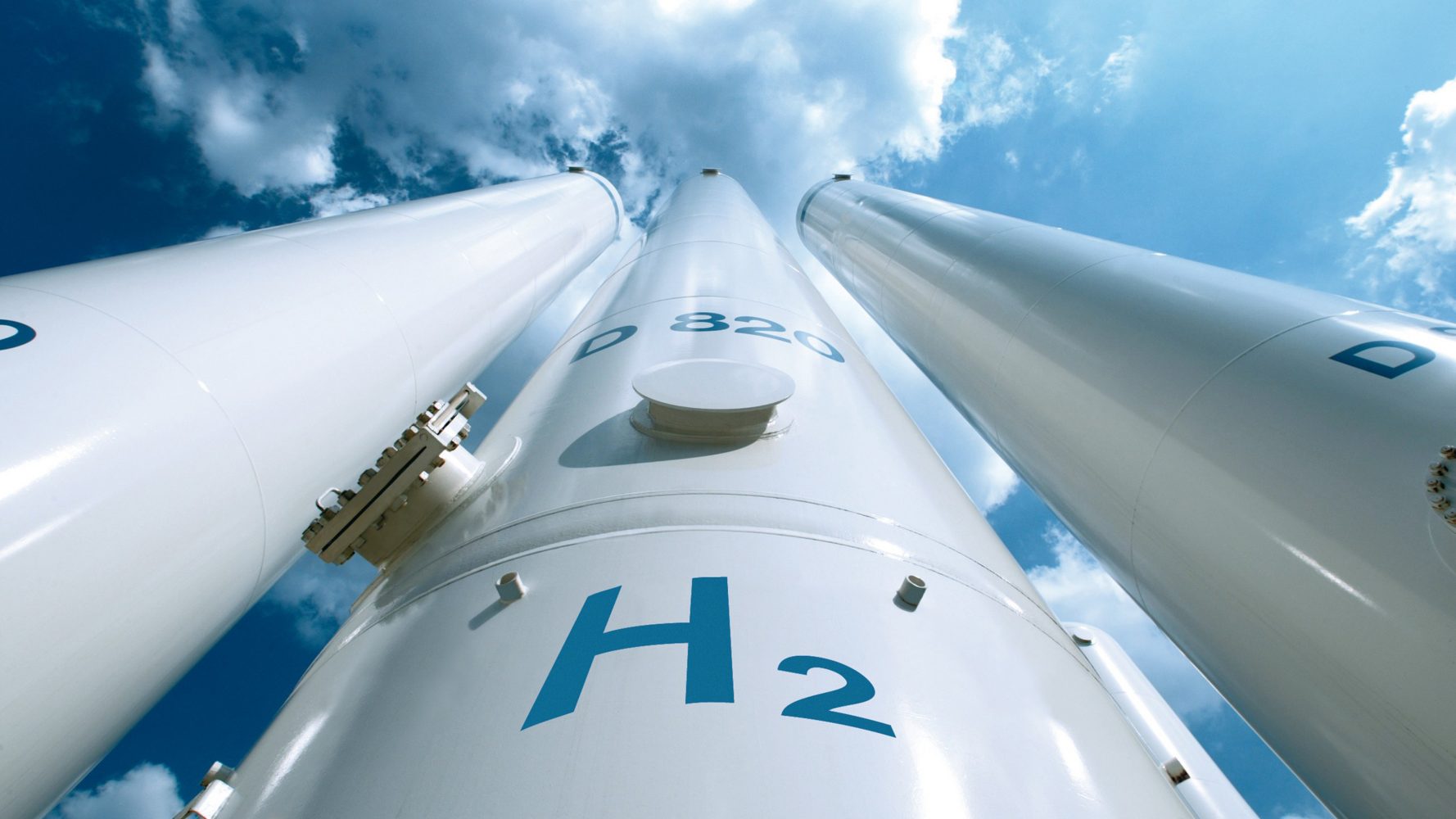
Era of Hydrogen
back to contentsThis year has become a milestone in the history of the hydrogen industry. Hydrogen technology has gone a long way from analyst forecasts and isolated attempts of individual companies to national and international strategies backed by solid funding. The pioneers in this field are the European Union and individual European companies.
Why hydrogen? Germany’s example
Germany is one of the European countries on the forefront of promoting the idea of carbon neutrality. On June 10, 2020, the Government of Germany adopted a National Hydrogen Strategy. However, why hydrogen?
One of the key reasons behind the choice of hydrogen is most likely the fact that massive expansion of renewable capacity in Germany has come to a halt, which became evident already in 2018. “In Germany, renewable capacity additions declined in 2018, but the reasons are not clear yet,” Alexander Korchagin, CEO of NovaWind (a wind power company and a subsidiary of Rosatom), said. In the first half of 2019, Germany added as little as 290 MW of new wind capacity, down 80% year over year. In 2018, new capacity additions decreased almost twofold as compared to 2017.
Why was it decided to quietly curtail investments in renewable energy sources? The main reason is that the bet on wind and sun as sources of energy generation capable of drastically reducing the volume of greenhouse gas emissions, did not pay off. It was not until 2018 that emission began to decline. Until then, they had been growing. It is a paradox, but emissions in Germany were declining in parallel with shrinking investments in new wind power capacity, which accounts for a major share in the total renewable capacity of the country.
The second reason is that the existing installed renewable energy capacity is already 25% higher than the stagnating demand for electricity. According to data from Energy-charts.de as of July 3, 2020, the total installed renewable capacity in Germany is 125.76 GW, while total consumption stands usually at 40 GW and rises to 80–100 GW at peak hours.
Third, renewable generation is unstable. “Caused by these changes, the secured power generation capacity in Germany will decrease from 87.2 GW in 2017 down to 54.8 GW in 2030, which will be for sure to less for a reliable energy supply of Germany with its peak load of 80–100 GW and based on its own capabilities,” Prof. Harald Schwarz, Chair of Energy Distribution and High Voltage Engineering at the Brandenburg University of Technology, writes in one of his articles.
The fourth reason is power price fluctuations and unbalanced distribution. “Unfortunately, renewable generation will often occur, when less energy will be needed. Therefore, several times per week the region distribution grids starts feeding back renewable overproduction to the overlaid 400 kV transmission grid,” Harald Schwarz continues.
Fifth, the grids are not developed enough to accommodate large and often unstable energy flows. The German National Energy Agency published a survey concluding that Germany needs to build 10,000 to 20,000 kilometers of 110 kV power transmission lines across the country. Prof. Schwarz notes, however, that only a few hundreds of kilometers of new lines were built over the last decade.
Sixth, there are no more vacant sites for solar or wind farms. Their proximity unnerves local residents and cause concerns about their health effects.
Seventh, expansion of renewables has driven up power prices. Officials have to promise there will be no further price growth.
And finally, the eighth reason, which has not been fully realized yet, is that there is no solution for the disposal of wind power towers as some of them have approached, or are approaching, the end of their service life.
Quick recap: Germany admits that the growth of renewable generation capacity has not addressed the existing challenges. Rather than reducing greenhouse gas emissions, renewables have made the energy supply less stable, changed landscapes and produced a new source of hi-tech waste.
The German experience is of course unique in its own way, but, at the same time, it is a warning to everyone who believes that renewable energy sources can be the basis of energy supply for a highly developed economy. Obviously, political considerations will not allow Germany to abandon renewable energy sources, but problems with the stability of energy supply must be somehow solved. Hydrogen economy has been declared a solution to the existing problems. And it seems there are no alternatives yet as no high-capacity power storage systems have been created and no new grids have been built.
Not just Germany
Other countries show interest in hydrogen economy as well. “Almost all Member States have included plans for clean hydrogen in their National Energy and Climate Plans; 26 have signed up to the Hydrogen Initiative, and 14 Member States have included hydrogen in the context of their alternative fuels infrastructure national policy frameworks. Some have already adopted national strategies or are in the process of adopting one,” authors of the European Strategy stated. Here are some examples.
In Spain, the latest edition of the alternative energy law designates hydrogen a key energy source. The Catalan Energy Institute has launched the Taula de l’Hidrogen project supported by 40 Spanish companies with the goal of promoting hydrogen technology.
In Russia, hydrogen is one of three components of the Roadmap for Development of the Power Storage Market in Russia.
In 2018, France adopted its Hydrogen Development Plan for Energy Transition, which will be included in the Long-Term Energy Program for 2019–2028.
The U.S. Department of Energy is ready to partially fund the installation of electrolyzers at a nuclear power plant operated by Exelon.
Private companies are also interested in hydrogen technology. The largest European energy companies and associations including Akuo Energy, BayWa r.e., EDP, Enel, Iberdrola, MHI Vestas, SolarPower Europe, Ørsted, Vestas and WindEurope have sent an open letter to the European Commission, pointing out that “Investment in renewable hydrogen has a great potential in terms of jobs and growth creation.”
Finally, the European Union has also developed a hydrogen strategy. Peculiarly, the strategy considers hydrogen to be ‘clean’ if it is produced with energy from renewable sources. The strategy reads, “‘Clean hydrogen’ refers to renewable hydrogen.” The experience of Exelon and, as we will see, Rosatom proves that carbon-free production of hydrogen is possible at nuclear power plants. Yves Desbazeille, Foratom Director General, spoke up for the role of nuclear in the hydrogen market, “Given the huge challenge Europe will face over the next 30 years, it is essential that policymakers do not focus only on variable renewables. Transforming our energy system is going to require ALL low-carbon solutions currently available. And the EU policy must reflect this.”
Who will pay for hydrogen?
It seems reasonable to assume that commitments of individual governments could be measured through allocated funds and announced project timelines. Exelon’s project and Germany’s strategy do have such measures.
The Exelon project is estimated at USD 7.2 million, with USD 3.6 million coming from the government. It is planned that the company will select an electrolysis site, complete 30% of the project, and simulate operation of the facility. The project is intended to diversify company’s business and increase revenue from the nuclear power plant. “Exelon has since 2018 been seeking ways to “re-purpose” its nuclear plants to make them more viable. The company’s efforts included convening academic experts, former employees, and former federal regulators in a brainstorm session. And over the last several years, what we have boiled that table down to is, basically, hydrogen. Hydrogen is what we want to look at going forward. We think it fits in with potentially a future hydrogen economy,” POWER Magazine quoted Exelon Nuclear Vice President for Engineering and Technical Support Scot Greenlee as saying.
As for Germany, the scope and sources of finance for the hydrogen economy are clearly detailed in the very beginning of its national strategy, “… between 2016 and 2026, a total of EUR 1.4 billion in funding will be provided. In addition to this, the Federal Government has made use of the financial resources provided under the Energy Research Program to build an excellent research landscape. Between 2020 and 2023, EUR 310 million will be provided under the Energy and Climate Fund for practice-oriented basic research on green hydrogen and there are plans to provide another EUR 200 million over this period to strengthen practice-oriented energy research on hydrogen technology. In addition, EUR 600 million will be provided between 2020 and 2023 to foster the ‘Regulatory Sandboxes for the Energy Transition’, which help speed up the transfer of technology and innovations from the lab to the market, not least for hydrogen solutions. As part of Germany’s decarbonization program, funding is provided for investment in technologies and large-scale industrial facilities that use hydrogen to decarbonize their manufacturing processes. More than EUR 1 billion will be provided for this between 2020 and 2023. There are also programs that promote the use of hydrogen in manufacturing and for the purpose of eliminating and utilizing carbon emissions in the base materials industry. These seek to encourage the industry to invest in hydrogen solutions. On June 3, 2020, the Coalition Committee adopted a ‘package for the future’ that makes available another EUR 7 billion for speeding up the market roll-out of hydrogen technology in Germany and another EUR 2 billion for fostering international partnerships. The precise amounts available for each of these programs depend on the budget estimates made by the responsible ministries.”
The investment section of the EU Strategy contains expert estimates of future investments. “From now to 2030, investments in electrolyzers could range between EUR 24 and EUR 42 billion. In addition, over the same period, EUR 220–340 billion would be required to scale up and directly connect 80–120 GW of solar and wind energy production capacity to the electrolyzers to provide the necessary electricity. Investments in retrofitting half of the existing plants with carbon capture and storage are estimated at around EUR 11 billion. In addition, investments of EUR 65 billion will be needed for hydrogen transport, distribution and storage, and hydrogen refueling stations. From now to 2050, investments in production capacities would amount to EUR 180–470 billion in the EU.”
Why not sooner?
On the one hand, the answer lies with the fact that only renewables have been seen as a silver bullet for growing emissions. On the other, hydrogen has inherent drawbacks, which became known almost a century ago. People have made many attempts at using hydrogen in different modes of transport, but they all failed for different reasons.
The first problem is that hydrogen mixtures with atmospheric oxygen are highly explosive and there are no safe and reliable storage systems. It is the explosion hazard that made airships a thing of the past, and this hazard has not gone away. In June 2019, an explosion destroyed a hydrogen fueling station in Sandvik (Norway). There were no casualties, but Uno-X, an owner of the station, suspended hydrogen sales at all of its three fueling stations in Norway, as well as in other European countries.
The second problem is high volatility of hydrogen and the smallest size of its atoms. Even a tiny leak in the tank will leave a vehicle without fuel. Just imagine that such vehicle is an aircraft. As a result, hydrogen storage systems (such as tanks consisting of nanotubes filled with hydrogen) are very expensive, and the total weight and size of a vehicle increase. Storing wholesale amounts of hydrogen looks even more challenging. Harald Schwarz proposes storing hydrogen in gas holders, but it is just a proposal.
The third problem is related to consumer experience. It is well known that transport is a driver of almost any industry. But the infrastructure for hydrogen-powered cars is less developed than the infrastructure for electric vehicles with lithium ion batteries. Due to the economy of scale, electric cars are cheaper, and the maximum distance between charging stations is longer.
The fourth problem is that commercial production of hydrogen requires huge amounts of water. Ultimately, water will not disappear, but producers will have to calculate water balance for each specific lake or river, from which water will be sourced.
And finally, the main problem of hydrogen, particularly in terms of decarbonization, is its origin and price. Steam methane reforming is the cheapest and therefore most common method of hydrogen production. Coal gasification is the oldest method, while electrolysis is the most environmentally acceptable but expensive technique. Hydrogen produced by electrolysis is considered to be ‘green’ (see the Terms and Definitions below). According to the experts working on the French national hydrogen strategy, the cost of hydrogen produced by electrolysis is EUR 4–6 per kilogram provided that electricity costs EUR 50 per MWh and the electrolyzer works 4,000-5,000 hours per year. For comparison, the cost of hydrogen produced by steam reforming is EUR 1.5–2 per kilogram. The authors of the strategy believe that the cost of electrolysis might fell to EUR 2–3 per kg. According to the EU Strategy, the cost of green hydrogen ranges from EUR 2.5 to EUR 5.5 per kilogram and is expected to decline due to the economy of scale.
Transportation is another factor affecting the cost. According to the French strategy, it increases the end price in such industries as food processing, metallurgy, electronics, and glass production to EUR 10–20 per kg. After transportation, the price of hydrogen rarely remains below EUR 8 per kg. One might assume that transportation would make hydrogen a local product, but Germany’s national strategy provides for a portion of green hydrogen produced to be imported through the northern (the North and Baltic Seas) and southern borders.
With government support and lobbying excluded, hydrogen is acceptable but not the most attractive source of energy. According to Rusatom Overseas (part of Rosatom), hydrogen cars are manufactured by only Toyota, Honda, Daimler, Nissan and GM, while electric cars are made by almost every global car manufacturer.
Trains are a relatively recent addition to the range of hydrogen-powered vehicles. The most successful experiment is Coradia iLint, a hydrogen train servicing a 600 km line in Lower Saxony. The technology was developed by LHB, now part of Alstom. The government of Lower Saxony plans to launch 14 more hydrogen trains by 2022. Such trains can travel up to 1,000 kilometers without refueling at a speed of up to 140 km/h. The first hydrogen train in the UK, Hydroflex, was launched in February 2020, but its tank is enough for only 75 miles. In June 2020, Alstom signed a five-year agreement with Snam, one of major energy infrastructure companies, to develop hydrogen trains for Italy.
Quick recap: creating a new sector in the energy industry requires large investments from stakeholders to make the new energy source cheaper. Without them, the hydrogen industry is most likely to fill a few particular niches, such as intercity passenger trains traveling for a distance of up to 1,000 kilometers.
Market specifics
Since the hydrogen market is driven by political will, it is not just production but also regulatory harmonization that needs to be stimulated. “Measure 13: Advocacy for an international harmonization of standards for mobility applications for hydrogen and fuel-cell-based systems (e.g. refueling standards, hydrogen quality, official calibration, hydrogen-powered car type approval, licensing for ships, etc.),” the German Hydrogen Strategy reads.
The draft version of the EU Strategy estimates the global hydrogen output at 74 million tonnes, and only 4% of this amount is ‘green’ hydrogen. Given the target price of EUR 1.5–2 per kilogram of hydrogen, it is easy to calculate that the current market size in monetary terms is about EUR 150 billion per annum. We will refrain from citing consumption and production forecasts because reality proved long-term forecasts futile.
Rosatom’s technology
Rosatom has been developing hydrogen technology and solutions for almost half a century.
OKBM Afrikantov, a large nuclear research and engineering center and a subsidiary of Rosatom, has developed a design for a nuclear power plant with an MGR-T reactor intended to generate electricity and produce hydrogen from different raw materials.
Leipunsky Institute of Physics and Power Engineering, also part of Rosatom, is working on liquid metal electrochemical hydrogen generators. Liquid metal is used here both as heat transfer medium and chemical agent. It works as follows. Water steam is supplied to a lower part of the vessel containing liquid metal and reacts with it. The reaction yields gaseous hydrogen, while oxygen remains dissolved in the melt. Heat-and-mass transfer in the melt intensifies, and hydrogen is easily separated from water, which has not reacted, in a condenser. The project is now in the research and development phase.
Another promising technology involves high-temperature helium reactors (HTHR) operating at 1,000°C. Test stands have been installed, and key components of the technology (reactor, ceramic fuel, energy conversion, equipment and structural materials) have been developed and tested.
As the global focus of attention began shifting towards hydrogen technologies in 2017, Rosatom included hydrogen economy into its corporate research and development strategy. Last autumn, the Russian nuclear corporation initiated a new project to launch a hydrogen-powered train service in the island of Sakhalin. This is a pilot project intended to test the technology and acquire necessary capabilities. The role of Rosatom is to supply fuel cells.
The project is advancing: in June 2020, Russian Institute for Nuclear Power Plant Operation (VNIIAES, a Rosatom company) signed a contract with Rosatom’s electric power division Rosenergoatom to conduct a feasibility study for a nuclear and hydrogen energy competence center to be established at the Kola nuclear power plant. “The purpose of the Competence Center will be validation of technology for the production, storage and transportation of electrolytic hydrogen. We are now at the very beginning of a long journey,” a representative of Rosenergoatom explained. VNIIAES will also develop technical requirements and design specifications for a hydrogen fueling station, a concept design of a cryogenic storage tank for medium and long-distance hydrogen transportation by sea, and a modular hydrogen liquefying unit. In addition, VNIIAES will develop a basis of design for a liquid organic carrier system for the hydrogen storage and transportation by ice-class tankers via Northern Sea Route to Japan.
The Kola NPP has two electrolyzers producing 20.5 and 21.33 cubic meters of hydrogen per hour. With the balance-of-plant needs amounting to 4,500 cu m per annum, it is easy to calculate that the existing capacity is more than enough to produce hydrogen on a commercial scale and sell it to customers. The electrolysis process is more efficient when combines with high-temperature steam. Its thermal energy replaces some of electric energy, thus improving the ratio of electricity consumed to hydrogen obtained. So it is quite likely that the study of VNIIAES will show how the existing technology can be improved.
Quick recap: Rosatom is engaged in the development of proprietary solutions for the hydrogen economy and continues building relevant competencies to win a share in the hydrogen market.
Hydrogen funding in Germany
Between 2016 and 2026:
- EUR 1.4 billion in funding will be provided
Between 2020 and 2023:
- EUR 310 million will be provided under the Energy and Climate Fund for practice-oriented basic research
- EUR 200 million is planned to be provided to strengthen practice-oriented energy research on hydrogen technology
- EUR 600 million will be provided to foster the ‘Regulatory Sandboxes for the Energy Transition’, which help speed up the transfer of technology and innovations from the lab to the market, not least for hydrogen solutions
- More than EUR 1 billion will be provided for investment in technologies and large-scale industrial facilities that use hydrogen to decarbonize their manufacturing processes
On June 3, 2020, the Coalition Committee approved:
- EUR 7 billion for speeding up the market roll-out of hydrogen technology in Germany
- EUR 2 billion for fostering international partnerships
- Precise amounts available for each of these programs depend on the budget estimates to be made by the responsible ministries
Hydrogen funding until 2030
under the EU Strategy
- EUR 24–42 billion will be invested in electrolyzers
- EUR 220–340 billion will be required to scale up and directly connect 80–120 GW of solar and wind energy production capacity to the electrolyzers to provide the necessary electricity
- Around EUR 11 billion will be invested in retrofitting half of the existing plants with carbon capture and storage
- EUR 65 billion will be invested in hydrogen transport, distribution and storage, and hydrogen refueling stations
From now to 2050, investments in production capacities will amount to EUR 180–470 billion in the EU.
Terms and definitions (from Germany’s National Hydrogen Strategy)
Grey hydrogen is based on the use of fossil hydrocarbons. Grey hydrogen is mainly produced via the steam reforming of natural gas. Depending on the fossil feedstock, its production entails considerable carbon emissions.
Blue hydrogen is produced using a carbon capture and storage (CCS) system. This means that the CO2 produced in the process of making hydrogen does not enter the atmosphere, and so the hydrogen production can be regarded on balance as carbon-neutral.
Green hydrogen is produced via the electrolysis of water; the electricity used for the electrolysis must derive from renewable sources. Irrespective of the electrolysis technology used, the production of the hydrogen is zero-carbon since all the electricity used derives from renewable sources and is thus zero-carbon.
Turquoise hydrogen is produced via the thermal splitting of methane (methane pyrolysis). This produces solid carbon rather than CO2. The preconditions for the carbon neutrality of the process are that the heat for the high-temperature reactor is produced from renewable or carbon neutral energy sources, and the permanent binding of the carbon.